MSI MPG 271QRX
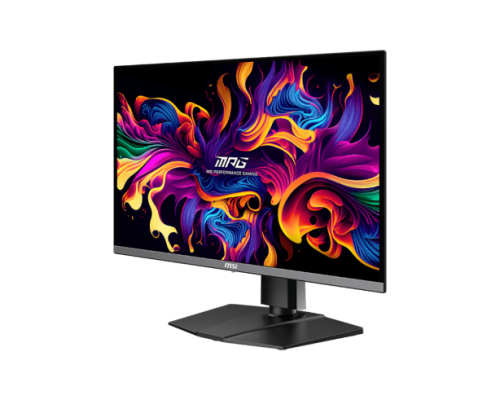
1 Specifications
Brand: | MSI |
---|---|
Model: | MPG 271QRX |
Size: | 26.5" |
Resolution: | 2560x1440 |
Panel type: | QD-OLED |
Max. refresh rate: | 360 Hz |
Panel: | Samsung SDC QMC256FF01_D01 |
Backlight type: | n/a |
Price (approx.): | USD 800 |
Monitor release date: | 2024-02 |
This review's date: | 2024-12 |
2 Other reviews
RTings.com review of the MSI MPG 271QRX.
TFTcentral review (mainly video) of the MSI MPG 271QRX.
3 At a glance
This monitor is the second OLED monitor reviewed for this website, after the ASUS PG27AQDP. Both monitors have the same screen size (26.5") and pixel resolution (2K) but come with different OLED technologies, the MSI being QD-OLED, the ASUS being WOLED. Both technologies differ, among other things, in the sub-pixel layout, QD-LED using a triangular layout vs. WOLED using a vertical stripe layout with 3 colors and white. Both layouts come with inevitable positional mismatch errors between the colors, and both are possibly incompatible with the assumptions made by operating systems about the sub-pixel layout when rendering text. This results in an artifact called color fringing – color seams along edges –, which is said to be more apparent with the QD-LED sub-pixel layout, but both monitors are worse in this regard than non-OLED monitors. That being said, the higher the pixel resolution – or, rather, the pixel density – the less of an issue the specific sub-pixel layout becomes. Both monitors have a pixel density of 111{unit|ppi}}, which certainly helps hiding such spatial artifacts.
Subjectively, the color fringing is indeed more apparent with the MSI than with the ASUS. Although this might be just because of the glossy screen finish of the MSI (vs. matte for the ASUS), it could also be because of the triangular sub-pixel layout being less optimal than the vertical stripe layout. The triangular layout provokes color fringing in both directions, X and Y, where color fringing in Y-direction might even be more problematic than in X-direction. Horizontal edges or horizontally aligned edge segments might be encountered more frequently (see letters) and/or are perceived differently than vertical edges (see left-right eye movements while reading). But this is just speculation.
The MSI is capable of a 360 Hz refresh rate (vs. 480 Hz for the ASUS), but for actually using such high refresh rate, sacrifices have to be made regarding color depth and/or signal compression. In contrast to the ASUS, the MSI does not make use of this high refresh rate internally for implementing a BFI mode (Black Frame Insertion) while being provided with an input signal of a lower and more manageable refresh rate. But the BFI implementation in the ASUS is flawed, so not much of an advantage for the ASUS here.
As probably most OLED monitors, also the MSI comes with a bunch of OLED care features which are intended to lower the risk of burn-in. All of these features can be disabled, except of what MSI calls pixel shift. Pixel shift causes the entire screen content to occasionally shift by one pixel at a time. However, this pixel shift frequency can be set as low as once per 12 minutes. Obviously, these features are implemented for a reason and should only be disabled if other measures against burn-in are in place, like operating the monitor in SDR mode (Standard Dynamic Range) at a moderate brightness.
Regarding color performance, a distinction should be made between nice and accurate, "nice" meaning well saturated colors across a wide range of viewing angles – which both monitors do provide – and "accurate" meaning colors being consistent with the received video data – which is where both monitors leave room for improvement – and colors being consistent with a color space model – which is where the MSI performs better than the ASUS. Both monitors are HDR-capable (High Dynamic Range) and are therefor able of processing 10 bpc input. Unfortunately, this does not mean that these monitors are also more accurate when processing 8 bpc signals, not even in the less demanding SDR mode (Standard Dynamic Range). This is a firmware issue which manufacturers should really be paying more attention to.
More a matter of personal preference is the screen surface finish and anti-glare coating (AG coating). Although the MSI has a glossy surface finish, it still has also same AG coating. According to the RTings.com review, the AG coating is even pretty good in reducing the overall reflection intensity. But the reflections appear nevertheless much less blurred than on the ASUS and its matte surface finish. Reflection coming from a QD-OLED screen are actually more tinted than they are on other screens – be it WOLED or non-OLED. But, honestly, this only becomes apparent when the reflections are so strong that their tint is the smallest problem.
4 Firmware
The monitor arrived with firmware version FW.009 and was updated to version FW.012 for this review. The current firmware version is shown, in faint contrast, at the bottom right corner of the on-screen display (OSD). For updating the firmware, the monitor must be connected via DisplayPort (i.e., not HDMI) and USB. Furthermore, a huge software package needs to be downloaded and installed (Gaming Intelligence utility, >500 MB). The update itself takes about 5 minutes.
On the one hand, being able to update the firmware is welcome – even the the update process seems to be unnecessarily cumbersome in this case –; on the other hand, this encourages manufacturers to release unfinished products and to make users beta testers. So, it is a double-edged sword.
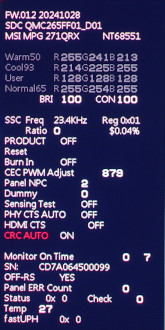
For showing the service menu, power off the monitor (via the left control button). Then, while pressing the mini joystick button, power the monitor on (again via the left control button) and keep the joystick pressed until the screen lights up. Once the monitor is fully up, press the joystick button to show the normal on-screen display (OSD). There will be a small "F" menu item above the normal OSD menu items – press the joystick button once more to select the "F", which brings up the service menu (see Figure 1). To close the service menu again, go "left" with the joystick. The "F" menu item will remain in the OSD until the monitor is power-cycled.
6 OLED care
OLED monitors are potentially susceptible to burn-in, meaning that static image content can alter pixel performance permanently when presented for a prolonged period of time. Manufacturers implement a number of counter-measures, almost all of which can be disabled in the case of the MSI MPG 271QRX, except for what MSI calls pixel shift. What also helps is to not present bright image content, where "bright" is construed relative to the maximum luminance in HDR mode, which is around 1000 cd/m2 for this monitor. Time will have to tell whether burn-in is still an issue when operating the monitor under such moderate conditions.

Pixel shift tries to avoid burn-in by occasionally shifting the entire screen around, by one pixel at a time. The user can choose how often this happens: "Slow" (once in 12 minutes), "Normal" (once in 8 minutes), and "Fast" (once in 4 minutes). Very occasionally, like once in 4 hours and for just one or two shift events in succession, the shift interval is only 1 minute. The screen is shifted mostly in diagonal directions, with direction reversals distributed somewhat randomly (see Figure 2). The random nature of the shift trajectory makes it difficult to determine the area that the trajectory can cover maximally and whether this area depends on the pixel shift timing. But, for example, the trajectory observed in "Fast" mode over a time period of 6 hours spanned 14x12 pixels.
7 Color saturation and accuracy

When it comes to color accuracy, one potential problem lies in the interactions between the primary color channels (red, green, blue) when rendering arbitrary colors, including white. Specifically for white, if everything was perfectly accurate, the luminances of the primary colors would add up to LW=LR+LG+LB(=LRGB for short). Deviations from this perfect relationship can be quantified by the (normalized) error Δe=(LRGB−LW) / LRGB (see Figure 3). We can interpret this error also as saturation error, de-saturation, or cross-talk coefficient. Note that, normally, the according measurements are also sensitive to de-saturation effects caused by the residual background illumination, which becomes overly dominant for dark shades. Dark shades are also more difficult to measure accurately, which is why they have been excluded from the graphs shown in Figure 3. Obviously, residual background illumination is not an issue with OLED monitors, because they don't have a backlight that would result in such illumination.
It is evident that the MSI performs much better in this test than the ASUS PG27AQDP. This is, most likely, due to the MSI being a QD-OLED monitor, where white is indeed created by mixing the color channels, whereas the ASUS is a WOLED monitor, where white is created by a dedicated white sub-pixel. Therefor, for a WOLED monitor, the observed error (Δe) is not a result of crosstalk between the colored sub-pixels, but is caused by a mismatch between the white-point of the white sub-pixel and the sum of the colored sub-pixels. Note that this has nothing to do with QD-OLED colors being possibly more saturated.
Normally, the Δe correlates with the more familiar dE color accuracy measure known from other review websites. For the MSI MPR 271QRX, the dE2000-value is 0.182 (averaged over 400 colors), which a little bit worse than for the Razer Raptor 27 165Hz (0.152, IPS monitor) but way better than the BenQ XL2540 (0.428, TN monitor) and, especially, the ASUS PG27AQDP (0.641, WOLED monitor). Figure 3 suggests that the MSI should have the lowest dE value in this contest, but the dE measure is potentially susceptible to color processing noise, which is where the MSI messes up.
8 Color processing noise
Ideally, the monitor processes incoming pixel values so that the according output luminance follows a smooth transfer function. This processing usually takes place in the digital domain, while aiming for some favorable Gamma characteristic and taking other parameters into account, like the Contrast setting, the RGB channel gains, and also spatial or spatio-temporal dithering. One way of quantifying how consistently this is done for all pixel values, without needing to know the specifically targeted Gamma characteristic, is to measure deviations from a smooth transfer function. This means that we do not focus on how well the transfer function is described by a simple Gamma function – this would be gamma tracking –, but how well the measured data points can be described by any reasonably smooth transfer function. We only measure the green channel here, because it is the brightest of the color channels and, thereby, provides the best signal-to-noise ratio. The lowest (x<16) and the highest pixel values (x>240) are not taken into account here, for several technical reasons related to the measurement method and data analysis.

Figure 4 shows the results for the MSI MPG 271QRX and, for comparison, the BenQ XL2540 which, although being a TN monitor, exhibits very low processing noise. Clearly, the MSI is doing much worse in this test than the BenQ (SD=19.9% vs. SD=6.3%; smaller standard deviations are better). And part of this noise is even repetition noise (as indicated by the curves below the y=0 axis in Figure 4). Note that the entire pixel value range was measured twice, and the repetition error is basically the absolute value of the difference between the two measurements, actually after a generous drift correction. This drift correction rules out any simple explanation for the high repetition errors. A comparison with a different OLED monitor, albeit not QD-OLED but WOLED (ASUS PG27AQDP, see Figure 5) rules out that this is a more general issue with OLED monitors, although the ASUS does not show particularly low repetition errors either.

Although the MSI, being an HDR-capable monitor, allows input signals with 10 bpc color depth, this does not appear to make a difference for this test (Figure 6), but the big repetition errors make this difficult to see. If the results were truly different, we would expect the 10 bpc results to be actually worse than the 8 bpc results, as explained in more detail in the Razer Raptor 27 165Hz review.
9 Settling behavior
The following measurements were made with a photo diode PDA36A (Thorlabs), the gain of which was set to 60 dB resulting in a bandwidth of 37.5 kHz and a minimal rise/fall time (10%-90%) of about 9 µs. The photodiode was placed at 4.5 cm from the screen surface at a straight angle. Ambient light was kept from the measured area by a rubber sleeve of 3 cm diameter which also limited the maximal incident angle to about ±20°.
The vertical extent of the measured screen area was not only limited by the rubber sleeve but also by the stimulus being a horizontal stripe covering just 5% of the screen height. Note that the OLED pixels are updated sequentially from the top of the screen to the bottom, which results in different delays for the luminance curves, depending on the vertical measurement position. By limiting the measurement to only 5% of the full vertical screen size, the smear effect caused by averaging over differently delayed luminance signals is limited to a known value. For example, at a refresh frequency of 120 Hz, the screen is updated within around 8 ms, so that a theoretically instant luminance onset on the single-pixel level would result in a measured luminance curve with a 0% to 100% transition ramp over a duration of 5%·8 ms = 0.4 ms. This smear effect is what limits the bandwidth of the measurement and determines, for example, the shortest rise/fall times that can be accurately measured this way. Normally, i.e., when measuring LCDs, this is not a limiting factor, because the LCDs switch considerably slower anyway. But with OLEDs, where the luminance onset – on the single-pixel level – can indeed be assumed to be instantaneous, this smear effect is what determines the measured rise/fall times, irrespective of the true rise/fall times being much smaller.
For more detailed information on the measurement method and the presentation of the results, see Flicker-free settling.
9.1 Settling curves
Figure 7 shows the luminance signal over time for the horizontal stripe being switched from black to white and back. Since the pixels in OLED monitors respond virtually instantaneously, the exact shape of the luminance transition is of minor interest. Nevertheless, Figure 7 still provides some noteworthy aspects.
- The latency (or input lag) is as short as can be expected, namely about half the duration of a refresh cycle. Note that these measurements were taken at the vertical screen center; therefore, and assuming that the pixels are updated right at the time they are received from the PC, half of the frame data had to be received when the update process arrives at the vertical screen center where the photodiode was placed at, which explains the measured latency of half a refresh frame cycle.
- The amplitude for the first refresh cycle is higher than for later refresh cycles – seemingly more so for 240 Hz than for 120 Hz –, as if the monitor was using overdrive. This is only the case for specific luminance values though, which we will see in the Settling matrix measurements section.
- Each refresh cycle ends (or, depending on how you look at it, starts) with a very brief low pulse. This is typical for OLED monitors and reflects the pixel reset phase that is part of the pixel refresh procedure. We cannot infer any precise per-pixel pulse timing from our simple measurements here, because the photodiode lumps together several pixels and pixel lines into one signal, thereby smearing out such short time events, as explained above. This is why the relative pulse amplitude is seemingly higher for 240 Hz than for 120 Hz; at double the refresh rate, the recorded pixel lines are simply updated twice as fast, which results in a smaller smear effect and thus in a less washed-out pulse (assuming the true pulse width stays the same).
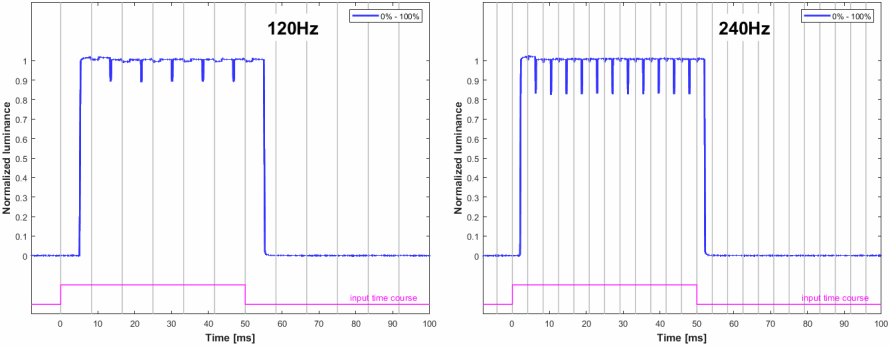
The vertical gray lines mark the times when the OpenGL command sequence SwapBuffers();glFinish(); returns control to the PC program, which is about when the 1st line of a frame is sent out to the monitor. This is also when, for the black to white frame switch, a hardware trigger is updated (pink trace), which is recorded along with the photodiode signal. The photodiode was placed at the vertical center of the screen.
9.2 Settling matrix measurements
The metrics typically presented in this section, such as settling times, fall/rise times, and similar measures, are intended to characterize the shape of the pixel response curves. However, for OLED monitors with their ultra-fast pixel response times, most of these measures have become largely irrelevant. Those that remain useful may need to be redefined to account for new signal features, such as the reset pulse, to ensure the measures are not contaminated by such features.
Figure 7, in particular, suggests that overshoot – or, alternatively, the average luminance error in the first refresh cycle after a pixel level change – might be worth looking at. Since luminance remains mostly constant throughout a refresh cycle, the average luminance error appears to be the more appropriate measure here, provided that sample time points potentially affected by the limitations of the measurement method are excluded. This also means that the pixel reset pulse is excluded from averaging.

The z-axis for the MSI matrix plots has been clipped at 6% to improve the scale for the values of interest. Note the different z-axis scales for the two monitors.
A "positive" error corresponds to the luminance being higher in the first refresh cycle than in later refresh cycles, no matter whether the FROM pixel value was larger or smaller than the TO pixel value.
To assess the aforementioned luminance error, the methods described in Flicker-free settling were used, with some modifications: the photodiode gain was set to 60 dB (instead of 70 dB), a low-pass filter frequency of 4 kHz (instead of 70 Hz) was applied to preserve the features of the fast OLED pixel response, and signal averaging was limited to an interval that is free of potentially contaminated samples, as outlined above. Note that, with these procedural adjustments, the signal bandwidth is ultimately constrained by the width of the measured stripe on the screen.
Figure 8 shows the luminance errors for the first refresh cycle after a pixel level change, for 120 Hz and 240 Hz, compared against the ASUS PG27AQDP. The large negative errors for the MSI are mainly caused by a slow rise time rather than by reaching a too low luminance in the first refresh cycle. In direct comparison to the ASUS, the MSI exhibits smaller errors for low-to-high pixel level changes (FROM < TO) but larger errors for high-to-low pixel level changes (FROM > TO). Except when switching between dark shades, the errors are mostly below 2% (for the MSI) and, thereby, way smaller than for LCD monitors, where anything below 20% would be considered above-average good already. Whether a 2% error holds any practical relevance or not, the observed error pattern raises questions about the underlying causes.
Could it be that the measured luminance deviations are actually not errors but compensations for, e.g., detrimental effects of the pixel reset pulse, similar to how overdrive in LCD monitors accelerates (and compensates for) slow rise and fall times? On the one hand, the reset pulse – on a single pixel level, i.e., not as measured here – is considered to be too short to have any relevant effect on the luminous output energy per refresh cycle; on the other hand, these pulses obviously carry enough energy to leave traces in our bandwidth-limited signal (see Figure 7). Note that the reset pulse is always negative, thereby giving high-to-low pixel level switches a slight advantage, regarding switching latency, over low-to-high switches, at least for the more extreme target levels (i.e., when switching from dark to bright or vice versa).

Here is another observation regarding the pixel reset pulse. Although we cannot measure the pulse width and amplitude on a single-pixel level directly, we still can infer the relative pulse energy from our bandwidth-limited measurements – at least for the settled portion of the pixel response curves –, namely by comparing two simple measures: the average luminance (LP) over the entire refresh cycle (i.e., including the pulse) and the average luminance (L0) over the refresh cycle excluding the pulse. The relative reset pulse energy e is then simply defined as e = 1 - LP / L0. If, on a single-pixel level, the pulse level is always zero and the pulse duration is constant (i.e., independent of the pixel level), then we would expect the relative pulse energy to be the same for all pixel levels. This, however, is not at all what has been measured – see Figure 9. Although the relative pulse energy scales nicely with the refresh rate, which is in line with the reasonable assumption that the pulse duration does not depend on the refresh rate, the relative pulse energy strongly depends on the luminance level. Mind you, this is just for refresh cycles where the pixel value remained unchanged; it is not for refresh cycles that involve an actual change in the pixel value. This is why we cannot draw any conclusions from this finding other than that the luminance settling behavior might be more affected by the reset pulse than expected – in whichever way. There is one caveat: inferring the pulse energy from a bandwidth-limited signal assumes that the measurement system is sufficiently linear. However, given that the MSI and the ASUS, which are two monitors with rather different OLED panels, appear to have such similar pulse energy signatures raises doubts regarding the validity of these measurements. Maybe these results just reflect some shortcomings of the measurement devices (photodiode, amplifier, oscilloscope) and/or the analysis method.
Back to the luminance error pattern. The luminance error, as a however defined measure, might largely depend on where exactly the refresh cycle is considered to start and how the effects of the signal bandwidth limitations are taken into account. There are a number of reasonable definitions for this error measure, and which one is the most appropriate might also depend on the use case one has in mind. One might be better for capturing luminance artifacts (e.g., of moved edges), another one might be better for capturing color distortions. For example, the start of the refresh cycle could be determined for each FROM-TO level transitions individually by aligning it with the 50%-point of the respective luminance transition edge, in contrast to using one absolute time point for all FROM-TO level transitions, which meets a certain criterion, like the 50%-point, only on average. Or, as another example, the pixel reset pulse could be either excluded from the luminance averages or not? Here, we chose to exclude the pulse, assuming that its impact is – if not negligible – at least the same for all level transitions. However, the findings summarized in Figure 9 suggest that both of these assumptions are likely wrong. Neither is the impact necessarily small (see dark levels), nor is the impact the same across different levels.
In conclusion, the results presented in this section should be interpreted with caution.
10 Color spectra